Introduction
Within This Page
Increased demands on the nation's electrical power systems and incidences of electricity shortages, power quality problems, rolling blackouts, and electricity price spikes have caused many utility customers to seek other sources of high-quality, reliable electricity. Distributed Energy Resources (DER), small-scale power generation sources located close to where electricity is used (e.g., a home or business), provide an alternative to or an enhancement of the traditional electric power grid.
DER is a faster, less expensive option to the construction of large, central power plants and high-voltage transmission lines. They offer consumers the potential for lower cost, higher service reliability, high power quality, increased energy efficiency, and energy independence. The use of renewable distributed energy generation technologies and "green power" such as wind, photovoltaic, geothermal, biomass, or hydroelectric power can also provide a significant environmental benefit.
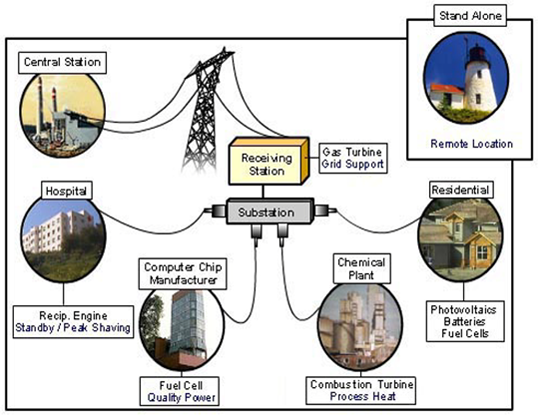
Fig. 1: Types of distributed energy resources and technologies
Image courtesy of the California Energy Commission
Description
A. DER Taxonomy
Distributed energy resources (DER) are electric generation units (typically in the range of 3 kW to 50 MW) located within the electric distribution system at or near the end user. They are parallel to the electric utility or stand-alone units. DER have been available for many years, and are known by different names such as generators, back-up generators, or on-site power systems. Within the electric industry the terms that have been used include distributed generation (DG), distributed power (DP), and DER. Note that the use of "DER" in this Resource Page refers to the broadest range of technologies that can provide power to the user outside of the grid, and includes demand-side measures.
Distributed Generation—Any technology that produces power outside of the utility grid (e.g., fuel cells, microturbines, and photovoltaics)
Distributed Power—Any technology that produces power or stores power (e.g., batteries and flywheels)
Distributed Energy Resources—Any technology that is included in DG and DP as well as demand-side measures. Under this configuration, power can be sold back to the grid where permitted by regulation.
B. Types of DER Technologies
DER technologies consist primarily of energy generation and storage systems placed at or near the point of use. Distributed energy encompasses a range of technologies including fuel cells, microturbines, reciprocating engines, load reduction, and other energy management technologies. DER also involves power electronic interfaces, as well as communications and control devices for efficient dispatch and operation of single generating units, multiple system packages, and aggregated blocks of power.
The primary fuel for many distributed generation systems is natural gas, but hydrogen may well play an important role in the future. Renewable energy technologies—such as solar electricity, biomass power, and wind turbines—are also popular.
The following table from the California Distributed Energy Resources Guide provides information regarding DER technologies that are commercially available as well as those still undergoing development. Some of the technologies are listed in both categories because they are currently commercially available but are also undergoing a significant level of further research and development.
DER Technologies | Commercially Available | Emerging Technology |
---|---|---|
Microturbines Microturbines are small combustion turbines that produce between 25 kW and 500 kW of power. Microturbines were derived from turbocharger technologies found in large trucks or the turbines in aircraft auxiliary power units (APUs). See also Microturbines. |
X | X |
Combustion Turbines Conventional combustion turbine (CT) generators typically range in size from about 500 kW up to 25 MW for DER, and up to approximately 250 MW for central power generation. They are fueled by natural gas, oil, or a combination of fuels ("dual fuel"). Modern single-cycle combustion turbine units typically have efficiencies in the range of 20 to 45% at full load. Efficiency is somewhat lower at less than full load. |
X | |
Internal Combustion Engines A reciprocating, or internal combustion (IC), engine converts the energy contained in a fuel into mechanical power. This mechanical power is used to turn a shaft in the engine. A generator is attached to the IC engine to convert the rotational motion into power. They are available from small sizes (e.g., 5 kW for residential back-up generation) to large generators (e.g., 7 MW). Reciprocating engines use commonly available fuels such as gasoline, natural gas, and diesel fuel. |
X | |
Stirling Engines Stirling engines are classed as external combustion engines. They are sealed systems with an inert working fluid, usually either helium or hydrogen. They are generally found in small sizes (1–25 kW) and are currently being produced in small quantities for specialized applications in the space and marine industries. |
X | |
Fuel Cells Fuel cell power systems are quiet, clean, highly efficient on-site electrical generators that use an electrochemical process—not combustion—to convert fuel into electricity. In addition to providing power, they can supply a thermal energy source for water and space heating, or absorption cooling. In demonstration projects, fuel cells have been shown to reduce facility energy service costs by 20% to 40% over conventional energy service. See also Fuel Cell Technology. |
X | X |
Energy Storage/UPS Systems Energy storage technologies produce no net energy but can provide electric power over short periods of time. They are used to correct voltage sags, flicker, and surges that occur when utilities or customers switch suppliers or loads. They may also be used as an uninterruptible power supply (UPS). As such, energy storage technologies are considered to be a distributed energy resource. |
X | X |
Photovoltaic Systems Photovoltaic (PV) cells, or solar cells, convert sunlight directly into electricity. PV cells are assembled into flat plate systems that can be mounted on rooftops or other sunny areas. They generate electricity with no moving parts, operate quietly with no emissions, and require little maintenance. See also Building Integrated Photovoltaic (BIPV). |
X | |
Wind Systems Wind turbines use the wind to produce electrical power. A turbine with fan blades is placed at the top of a tall tower. The tower is tall in order to harness the wind at a greater velocity, free of turbulence caused by interference from obstacles such as trees, hills, and buildings. As the turbine rotates in the wind, a generator produces electrical power. A single wind turbine can range in size from a few kW for residential applications to more than 5 MW. |
X | |
Hybrid Systems Developers and manufacturers of DER are looking for ways to combine technologies to improve performance and efficiency of distributed generation equipment. Several examples of hybrid systems include:
|
X |
C. Characteristics of DER
Some of the primary applications for DER include:
- Premium power—reduced frequency variations, voltage transients, surges, dips, or other disruptions
- Back-up power—used in the event of an outage, as a back-up to the electric grid
- Peak shaving—the use of DER during times when electric use and demand charges are high
- Low-cost energy—the use of DER as base load or primary power that is less expensive to produce locally than it is to purchase from the electric utility
- Combined heat and power (cogeneration)—increases the efficiency of on-site power generation by using the waste heat for existing thermal process
Generally, DER provides the consumer with greater reliability, adequate power quality, and the possibility to participate in competitive electric power markets. DER also have the potential to mitigate overloaded transmission lines, control price fluctuations, strengthen energy security, and provide greater stability to the electricity grid.
Accessible: N/A
Aesthetics
- Improves sightlines and views with off-the-grid systems, which eliminate the need for overhead power lines
- Gives more choice in energy supply options; allows customers to choose the best solution for an individual location
Cost-Effective
- Enables cost savings by reducing the peak demand at a facility, therefore lowering demand charges
- Provides greater predictability of energy costs (lower financial risk) with renewable energy systems
- (See Section F: Economics of DER)
Functional
- Provides better power reliability and quality, especially for those in areas where brownouts, surges, etc. are common or utility power is less dependable
- Facilitates DER equipment efficiency improvements when used in combination with combined heat and power equipment for heating, cooling, and dehumidification applications
- Provides power to remote applications where traditional transmission and distribution lines are not an option. Locations such as cellular towers, small remote towns, or drilling platforms in the ocean are outside the electric grid and benefit from DER as a primary power source
- Possesses combined heat and power capabilities
- Reduces upstream overload of transmission lines
- Optimizes utilization of existing grid assets—including potential to free up transmission assets for increased wheeling capacity
- Improves grid reliability
- Facilitates faster permitting than transmission line upgrades
- Provides ancillary benefits—including voltage support and stability, contingency reserves, and black start capability (ability of a generating unit during a system restoration to go from a shutdown condition to an operating condition and start delivering power without assistance from the electric system)
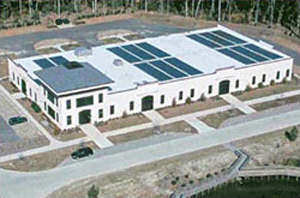
Fig. 2: This photovoltaic installation, located in a showcase eco-industrial park, will prevent more than 6,000 tons of pollutants from being released into the air over its lifetime. Cape Charles Sustainable Technology Park—Cape Charles, Virginia
Image courtesy of PowerLight Corp.
Productive
- Some DER equipment provides high quality power for sensitive applications
- Responds faster to new power demands—as capacity additions can be made more quickly
- Facilitates less capital tied up in unproductive assets—as the modular nature of distributed generators means capacity additions and reductions can be made in small increments, closely matched with demand, instead of constructing central power plants sized to meet estimated future (rather than current) demand
- Back-up power decreases downtime, enabling employees to resume working
Secure/Safe
- Strengthens energy security
- Back-up power provides quick recovery after an event
Sustainable
- Provides cleaner, quieter operation, and reduces emissions for some technologies (e.g., solar, wind, fuel cells)
- Reduces or defers infrastructure (line and substation) upgrades
- Possesses higher energy conversion efficiencies than central generation
- Enables more effective energy and load management
D. Characteristics of DER Technologies
Technology | Benefits | Drawbacks | Fuel Choice | Size (kW) |
---|---|---|---|---|
Microturbines Efficiency is 28% to 33% |
· Thermal recovery improves efficiency · Thermal output for residential or small commercial apps · Operable as base, peaking, or back-up · Commercially available in limited quantities |
· Insufficient thermal output for industrial apps | Natural gas, propane, diesel, multi-fuel | 15–250 |
Small Gas Combustion Turbines Efficiency is 25% to 40% |
· Highly efficient when used with thermal recovery · Technology commercially available today—most likely candidate for on-site needs >3 MW in DG application · Can operate base load, back-up, or peaking · Several manufacturers · Relatively low installed costs |
· Potentially onerous siting and permitting requirements · Environmental issues—emissions and noise · Possible on-site fuel storage needs |
Natural gas, distillate, methane | 3,000–15,000 |
Internal Combustion Engines Efficiency is 28% to 37% |
· Bulk power delivered when utility is unavailable · Fast startup allows less sensitive processes to be served without need for UPSs (emergency lighting, HVAC, elevators, some manufacturing processes) · Very mature, stable technology · Can be paralleled to grid or other generators with controls package · Can be very efficient when combined with heat recovery |
· Insurance policy effect: Capital is only being used when back-up generator is running · Marginal cost of production generally favors utility source in all but rare occasions · Environmental issues—emissions and noise · Possible on-site fuel storage needs |
Diesel, natural gas, propane, bio-gas, other petroleum distillates | <1–6,000 |
Fuel Cells High temp: Efficiency is 45% to 55% Low temp: Efficiency is 30% to 40% |
· Very high fuel efficiencies from hydrogen to electricity · Potential to operate base load with utility back-up · Possible residential application—a no-moving-parts energy appliance · Very high efficiencies when combined with heat recovery · Green technology—water and heat are only emissions from hydrogen fuel, low emissions from other fuels |
· Few commercially available devices · Most research efforts are for automotive applications · Need for fuel reformer in almost all applications (reduced fuel to electrical efficiencies) · Not a zero-emission technology—the effect of that may vary by state · Cold start is 1-2 days for MCFC, 3 hours for PAFC, 1 hour for PEMFC, and 2 minutes for SOFC |
Direct by hydrogen; natural gas, propane, methanol, or other hydrogen-rich source through reformer | MCFC (molten carbonate fuel cells): 250–2,850 PAFC (Phosphoric acid fuel cells): 200 PEMFC (proton exchange membrane fuel cells): 3–250 SOFC (solid oxide fuel cells): 225–2,240 |
Photovoltaic | · No variable costs for fuel · No moving parts—inexpensive maintenance and long life · No emissions, no noise · Can be used for peak shaving · Highly reliable, mature technology |
· Big foot print (600 ft²/kW) · High installed costs · Not suited for base load · Not suited for back-up except when accompanied by storage · Variable energy output |
None | Limited by available area |
Large Wind Turbines | · No variable costs for fuel · In utility implementation, zero emissions may allow green power price premium · Mature technology · Multiple manufacturers |
· Need to meet siting requirements · Generation is intermittent with wind, and energy output can vary with wind speed squared or cubed over operation range. Not appropriate as backup or off-grid applications · Needs utility source for energy purchases and sales · Can require footprint up to 100ft²/kW |
None—need winds of >12 mph or sometimes higher | <1–1,000 |
Source: Andersen, Reprinted from Public Utility Reports, Inc., from the Summer 2001 issue of Fortnightly's Energy Customer Management
E. Getting Started with DER
It is important to thoroughly understand the relevant issues related to DER before installing any DER technology/equipment. The following general steps will help one get started with DER:
- Clarify the reasons for wanting DER
- Identify the current and future technology options
- Evaluate the cost and savings
- Understand the regulations and project development process
- Understand the risks and uncertainties.
The following table from the California Distributed Energy Resources Guide summarizes the types of questions that need to be answered when purchasing DER equipment that will meet the energy requirements at an acceptable cost.
Decision Analysis Questions | Cost of Electricity Variables |
---|---|
APPLICATION | |
Residential, commercial, or industrial? Base load, backup, or peak shaving? Grid independent or grid parallel? |
Capacity Factor (CF) |
TECHNOLOGY | |
PEMFC, SOFC, ICE, CT, PV, Wind? Average electric load? Ideal power rating of the DER system? Heat rate of the DER system? Reliability of the DER system? Capital cost of the DER system? Installation cost of the DER system? O&M cost of the DER system? Method of payment for the DER system? DER system life? |
Fixed Charge Rate (FCR) Total Installed Cost (TIC) Operation & Maintenance Cost (O&M) Average Annual Net Plant Heat Rate(NPHR) |
FUEL | |
Natural gas, propane, or diesel? | Natural Gas Price (NGP) Diesel Oil Price (DOP) Propane Price (PP) |
F. Economics of DER
Cost is an important factor when considering the purchase of any product, including a DER technology. However, determining the cost of a DER technology is often more complex than simply purchasing a piece of hardware at a published price. In addition to equipment (or capital) cost, there are labor and other expenses related to installing the equipment. The cost of electricity produced by the DER technology can also be estimated and compared to the price currently being paid for electricity from the power grid.
Capital Costs of Selected DER Equipment | O&M Costs of Selected DER Equipment | ||
---|---|---|---|
DER Equipment | Capital Cost ($/kW) | Time Until Maintenance Required (hours of operation) | Average Maintenance Costs (¢/kWh) |
Microturbines | 700–1,100 | 5,000–8,000 | 0.5–1.6 (estimated) |
Combustion Turbines | 300–1,000 | 4,000–8,000 | 0.4–0.5 |
Internal Combustion Engines | 300–800 | 750–1,000: change oil and oil filter 8,000: rebuild engine head 16,000: rebuild engine block |
0.7–1.5 (natural gas) 0.5–1.0 (diesel) |
Fuel Cells | 700–1,100 | Yearly: fuel supply system Yearly: reformer system check 40,000: replace cell stack |
0.5–1.0 (estimated) |
Photovoltaics | 4,500–6,000 | Biyearly maintenance check | 1% of initial investment per year |
Wind Turbines | 800–3,500 | Biyearly maintenance check | 1.5–2% of initial investment per year |
Application
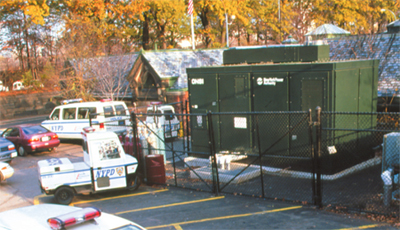
Fig 3. Fuel cells installed at a police station in NYC's Central Park.
Image courtesy of UTC Fuel Cells
Users of DER have different power needs. Hospitals need high reliability (back-up power) and power quality (premium power) due to the sensitivity of equipment. Industrial plants typically have high energy bills, long production hours, and thermal processes, and would therefore seek DER applications that include low-cost energy and combined heat and power. Computer data centers require steady, high-quality, uninterrupted power (premium power). DER technologies are available now and are being developed to meet these needs.
DER projects include:
-
A police station in New York's Central Park bought a fuel cell to run its electronic crime-fighting equipment, saving $200,000 over the cost of a line upgrade. More...
-
The Shop 'n' Save supermarket chain has installed base load generators at almost a dozen of its grocery stores, avoiding $40,000 in losses per day in the event of a power outage.
-
The Santa Rita Jail in Dublin, California has an on-site photovoltaic system that produces 1.18 megawatts, giving $15 million in net savings over the 25–year life of the project. More...
- A natural gas distribution company serving over 240 communities in Minnesota installed a natural gas powered microturbine in a cogeneration project to power the plant's liquefaction section and supply heat in the winter and cooling in the summer. More...
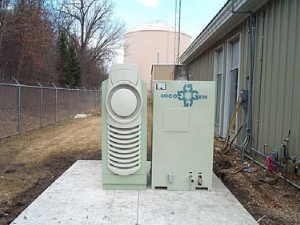
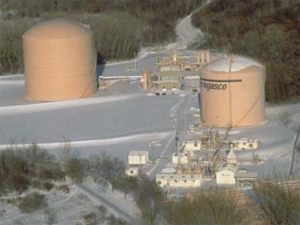
30 kW Capstone Microturbine installed at a natural gas distribution company.
Image courtesy of Capstone
Microturbines are used at a natural gas distribution company for power and space heating and cooling.
Image courtesy of Capstone
Relevant Codes and Standards
- Energy Policy Act of 2005 (EPACT)
- Executive Order 13693, "Planning for Federal Sustainability in the Next Decade"
- National Energy Policy. Report of the National Energy Policy Development Group, May 2001. Encourages "increasing energy conservation and efficiency" and "increasing America's use of renewable and alternative energy."
- IEEE Standards Association IEEE 1547a - Standard for Interconnecting Distributed Resources with Electric Power Systems, Amendment 1
- IEEE Standards Association IEEE Std 929-2000 Recommended Practice for Utility Interface of Photovoltaic (PV) Systems
- NFPA 70 National Electrical Code®—Contains provisions for installing photovoltaics.
Additional Resources
State and Federal Agencies
Associations and Organizations
- American Solar Energy Society (ASES)—A national organization dedicated to advancing the use of solar energy for the benefit of U.S. citizens and the global environment. ASES promotes the widespread near-term and long-term use of solar energy.
- CHP Association—An association that brings together diverse market interests to promote the growth of clean, efficient CHP in the United States. Its mission is to create a regulatory, institutional, and market environment that fosters the use of clean, efficient CHP as a major source of electric power and thermal energy in the U.S.
- Fuel Cell & Hydrogen Energy Association (FCHEA)—A trade association for the fuel cell and hydrogen energy industry dedicated to the commercialization of fuel cells and hydrogen energy technologies. FCHEA represents the full global supply chain, fuel cell materials, components and systems manufacturers, hydrogen producers and fuel distributors, automobile manufacturers, national laboratories, trade associations, and others.
- Solar Energy Industries Association (SEIA)—The national trade association of solar energy manufacturers, dealers, distributors, contractors, and installers. SEIA's primary mission is to expand the use of solar technologies in the global marketplace.
- World Alliance for Decentralized Energy (WADE)—Launched by a group of major companies and national industry associations to accelerate the development of high efficiency cogeneration and decentralized energy (DE) systems which will deliver substantial economic and environmental benefits worldwide.
Publications
- Distributed Energy Resources and Combined Heat and Power: A Declaration of Terms by Anna Monis Shipley and R. Neal Elliott. April 2000
Design and Analysis Tools
- HOMER Energy (Hybrid Optimization of Multiple Energy Resources)—A computer modeling software developed by NREL to help design stand-alone electric power systems. Users enter information about electrical loads, renewable resources, and component costs, and HOMER designs the optimal power system to serve those loads.