by Richard A. Hays, for the Director, Corrosion Policy & Oversight (DCPO), (DASD) [Materiel Readiness]
Updated: 10-07-2021
INTRODUCTION
Within This Page
Corrosion is most often associated with "rust" on steel and the oxidation of other metals. However, 10 U.S.C. § 2228 defines corrosion as, "the deterioration of a material or its properties due to a reaction of that material with its chemical environment." It is inclusive of the deterioration of all materials, which can be caused through moisture, sun exposure, mold and mildew, wind, and other environmental elements.
DESCRIPTION
Understanding the scope of this definition requires an explanation of the "Science of Corrosion." Other sections of the WBDG CPC Source explain the impacts and possible solutions for the control of corrosion. Photos 1 and 2 provide two vivid examples of corrosion on metals. Further explanation will be addressed in this Corrosion Science Page.
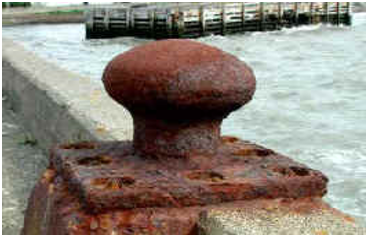

Photos 1 and 2: Advanced metallic corrosion.
Photo credit: D, CPO
Environmental Severity Factors Influencing Corrosion
There are several environmental severity factors that influence corrosion. These include but are not limited to:
- Temperature
- Time of wetness and humidity
- Presence of chlorides
- pH
- Industrial pollutants
- UV radiation
- Microbiological activity
These factors influence the degradation of materials in multiple ways. None are the same, but the impact, unless mitigated, can be devastating. Corrosion affects metals, wood, pavements, fabrics, roofs, and building systems. The International Standards Organization (ISO) has a straight-forward approach to categorizing a region's corrosivity. As shown on the ISO Corrosivity Map in Figure 1, coastal areas tend to represent a higher corrosion threat due to the presence of chlorides and moisture in the presence of heat.
While this is a good start to understanding the risks associated with environmental severity factors, local area considerations of climate, industrial impacts and intense solar exposures may result in higher corrosion rates than one would expect from only the presence of chlorides. It is prudent to investigate and understand environmental severity impacts for specific building and construction sites to establish the local corrosivity risk. See the Environmental Severity Page for a more extensive explanation related to this risk area.

Figure 1: ISO Corrosivity Categories (U.S. ICCET Corrosivity Heat Map)
Photo credit: Map Data © 2016 Google, INEGI
Interior Environments and Corrosion
In addition to external environmental factors, certain corrosion prone locations exist for building interiors and interiors of piping systems and tanks.
-
High humidity interior areas (for example, bathrooms, locker rooms, laundry rooms, pools, and trainers)
-
Interior areas open to the exterior (for example, mechanical rooms and hangars), and spaces that are not conditioned by design or may not be conditioned during prolonged periods due to deployment or occupancy.
-
Interiors of piping systems and tanks. Conditions such as pH (both acidity and alkalinity), dissolved oxygen), ferric scale, flow conditions, biological activity (microbiologically influenced corrosion), and the presence of disinfectants and corrosion inhibitors can all affect internal corrosion.
The Electrochemical Cell and Corrosion
Metallic corrosion results from the formation of an electrochemical cell. There are four fundamental components in an electrochemical corrosion cell: An anode, a cathode, an electron path (electrical connection) between the anode, cathode, and a conducting environment (electrolyte [see Figure 2]). All four components must be present for corrosion to occur. Metal at the anode is oxidized, causing it to form a metal oxide, that is, to corrode. The electrons that are released as a result of this reaction travel to the cathode through the metallic path. The over-abundance of electrons at the cathode prevent it from corroding. The most common electrolyte mediums are water, soil, and chloride-contaminated concrete. The electron path is an electrical connection, usually with some amount of resistance. It can be internal to the metal itself or external such as a wire, direct connection, or other interconnecting metallic components.

Figure 2: Basic Corrosion Cell
Diagram credit: D, CPO
The forms of corrosion in a given system depend on the materials, configuration, operation, and environment. The types and extent of cathodic protection, coating, etc. depend upon the corrosion risk factors discussed in this webpage. See the CPC Source Knowledge Area - Cathodic Protection for more information.
Identifying and Recognizing Common Forms of Corrosion
The common forms of corrosion include (see Table 1 and Photos 3 thru 7):
- Uniform (General) corrosion
- Pitting
- Crevice
- Galvanic corrosion
- Erosion corrosion
- Intergranular
- Selective leaching/dealloying
- Stress corrosion cracking
- Solar ultraviolet degradation
- Other less frequent forms (e.g., exfoliation, environmentally assisted cracking, corrosion fatigue)
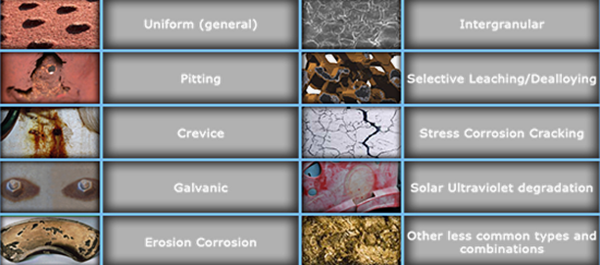
Table 1: Common Depictions of Corrosion
Photo credit: D, CPO
Here is a summary description of the major forms of corrosion in almost any locale regardless of the environmental severity of the location:
-
Uniform (general) is a form of corrosion that occurs uniformly over the entire exposed surface of a metal. Applying a barrier coating is one of the most common methods to mitigate this degradation
-
Pitting is a form of localized corrosion that occurs when a corrosive medium attacks a metal at specific points and results in deep cavities in the metal
-
Crevice is a form of corrosion that occurs when an electrolyte becomes trapped and stagnant in particular locations such as in joints, in corners, and under debris. It can be hard to find and extremely aggressive, causing high pitting rates and subsequent unexpected failures
-
Galvanic corrosion results from the formation of a galvanic cell by the galvanic coupling of dissimilar metals (metals having different electrical potentials), which are exposed to an electrolyte. This form can be very aggressive, but it may also be one of the easiest to detect and prevent
-
Erosion corrosion is due to the increased rate of deterioration and loss of a material from the combined effects of corrosion and the repeated motion of the surrounding environment. It is most often found in high-velocity areas or in fluids containing abrasive materials
-
Intergranular is a form of corrosion that attacks grain boundaries in materials. It may occur as a result of a galvanic couple between differing phases within a material and may be prevented by avoiding susceptible alloy or heat treatments
-
Selective leaching or dealloying is a localized form of corrosion where a particular constituent within a material is preferentially attacked and extracted from the material. Many copper alloys such as brasses and bronzes are susceptible alloys to this attack
-
Stress corrosion cracking (SCC) is a cracking process involving the combined factors of a susceptible material, corrosive environment and a sustained tensile stress
-
Solar ultraviolet degradation is the process by which organic-based polymers undergo photolytic and photo-oxidative reactions during exposure to solar UV radiation
-
Other types of corrosion:
-
Environmentally Assisted Cracking is a combination of a tensile stress, a corrosive environment, and a susceptible material. Stress disrupts protective films at crack tips. The corrosion process generates atomic hydrogen that reduces cracking resistance of material. EAC usually occurs on very-high-strength materials such as fasteners
-
Corrosion Fatigue is a process in which fatigue resistance of most materials is reduced in the presence of a corrosive environment. Corrosion processes that occur at crack tips increase the rate of fatigue crack growth. It occurs in structural materials such as aluminum and steel
-
Alkali-silica reactions on concrete occur over time in concrete between the highly alkaline cement paste and the reactive non-crystalline (amorphous) silica found in many common aggregates, given sufficient moisture. This reaction causes the expansion of the altered aggregate causing spalling and loss of strength of the concrete, finally leading to its failure
-
-
Environmental factors that increase corrosion risk:
-
Mold requires four factors to be present (mold spores, organic matter [like wood, paper, and drywall], moisture, and warmth) in order to grow or become established. See Mold and Moisture Dynamics, UFCs and UFGSs that address the various aspects of designing and maintaining to prevent and mitigate the impacts of mold. For example, see the applicable sections of UFC 3-101-01 Architecture for more information.
-
Soil resistivity, moisture content, and pH:
-
Soil resistivity and moisture content has the greatest impact on corrosion with respect to soil properties. Soils such as clays with the poorest drainage typically have the highest moisture content and lower resistivity values and are generally the most corrosive.
-
More acidic soils (pH 0—4.5) may represent a serious risk to common construction materials and metal (soils usually have a pH range of 4.5—8.0)
-
Soil acidity is produced by mineral leaching, decomposition of acidic plants, industrial wastes, acid rain and certain forms of micro-biological activity
-
-
Chlorides, sulphides, & bacteria:
-
Chloride ions are harmful, as they participate directly in pitting initiation of metals and their presence tends to decrease the resistivity of electrolytes including soils. They may be found naturally in soils as result of brackish groundwater and historical geological sea beds or from external sources such as de-icing salts
-
Sulfates are generally considered to be less aggressive; however, sulfates can be converted to highly corrosive sulfides by anaerobic sulfate-reducing bacteria. These microorganisms normally operate in temperatures from 20 to 30°C, pH from 6 to 8, and soil resistivities from 500 to 20,000 Ω
-
Bacteria are ubiquitous and their presence can influence the initiation and/or propagation of corrosion. Fuel-storage and fuel-handling systems are particularly susceptible to microbiologically influenced corrosion (MIC). See CPC Source, Petroleum, Oil, and Lubricants (POL) Storage and Distribution Systems Knowledge Area for more specific insights into the impacts of MIC on POL systems.
-
-

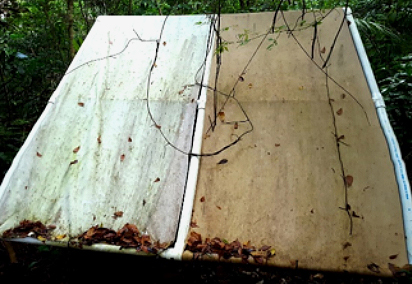
Photo 3: General and crevice corrosion of steel
Photo Source: D, CPO
Photo 4: Degradation of fabrics in a tropical environment
Photo Source: D, CPO
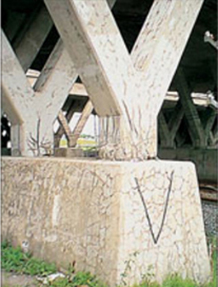

Photo 5: Alkali-silica reaction in concrete
Photo Source: D, CPO
Photo 6: Environmentally influenced cracking
Photo Source: D, CPO

Photo 7: UV degradation of organic coating systems
Photo Source: D, CPO
Conclusion
It is important to recognize the types of corrosion as well as the susceptibility of the materials where corrosion can occur. Knowledge of corrosion science, materials and the ability to recognize the signs of corrosion will assist in developing a mitigation strategy and pursuing associated actions that will serve to reduce life-cycle costs and extend facility durability and sustainability.
Here are a few examples of associated actions that will reduce life cycle costs and help improve facility durability:
- Design structures to minimize pooling of water
- Isolate galvanically dissimilar metals from one another
- Avoid using aluminum alloys in highly alkaline and highly acidic environments
- Coat steels to protect them from chloride-containing media such as seawater and deicing materials
- Apply cathodic protection to protect immersed or buried steel structures
- Add UV blockers to protect organic materials such as coating systems and fabrics in high solar exposures
- Use dehumidification in marine and tropical areas
- Mitigate against the impacts of heat and chlorides in association with other corrosion-impacting factors
- Use treated and coated wood products if they will be in contact with soils or in waterfront environments
- Use coatings appropriate for the intended use to protect structures
- Apply proper construction and maintenance practices, accompanied by appropriate material selection and surface treatments, to reduce the impacts of ultraviolet degradation on asphaltic materials in pavements and roofs
- Use appropriate concrete cover and coatings to minimize spalling in reinforced concrete structures
The CPC Source provides additional knowledge and practical information to help the Architect, Engineer, Facility Management and Acquisition professionals make the right corrosion related decision. The relevant UFC and UFGS criteria will provide insights and guidance on the best approach to mitigate the impacts of corrosion and the associated environmental factors increasing those risks.
Relevant Codes and Standards
International Organization for Standardization (ISO)
- ISO 9223:2012 Corrosion of metals and alloys—Corrosivity of atmospheres—Classification, determination and estimation
- ISO 9226:2012 Corrosion of metals and alloys—Corrosivity of atmospheres—Determination of corrosion rate of standard specimens for the evaluation of corrosivity
- ISO/TC 156/WG4 ISOCORRAG International Atmospheric Exposure Program: Summary of Results
Additional Resources
Publications
- A Cumulative Damage Approach to Modeling Atmospheric Corrosion of Steel by David H. Rose, Dissertation - University of Dayton. December 2014.
- Facilities Environmental Severity Classification Study Report by Leidos. February 16, 2017.
- CPC Source—Environmental Severity Classification (ESC)
- Corrosion Toolbox
- CPC Checklists Tool
Education
- WBDG: Continuing Education (DoD) (Login Required)
- Facilities Corrosion Knowledge Track Summary
- CPC Source Training
- Defense Acquisition University
- NACE (AMPP) website
- SSPC (AMPP) website
DoD Installations Organizations