Introduction
Within This Page
- Introduction
- Description
- Application
- Operation and Maintenance
- Special Considerations
- Additional Resources
THIS PAGE SUPPORTED BY THE
The wind industry has had an interesting history. Specifically, wind technology has evolved immensely, from hundreds of years ago when the Chinese and Danish harnessed wind energy to move water or grind grain, to the 1930s, when wind was used to provide electric power to Midwestern farmers and ranchers before the 1936 Rural Electrification Act. The technology has continued to evolve and become more important over time in response to volatile fossil fuel prices and global climate change. The concept of wind energy may be simple—wind rotates a rotor, the rotor rotates a shaft, and the shaft powers a generator. But wind energy can take many forms, and there are many different segments of the industry that serve different needs.
According to the American Wind Energy Association, at the end of 2010, the U.S.-installed wind capacity topped 40,000 megawatts (MW). In the previous four years, wind accounted for more than 35% of all new generation capacity in the U.S., second only to natural gas. Most installed wind capacity in the United States is currently in utility-scale wind farms, a term that refers to a group of a multiple wind turbines that has the capacity to reach utility-scale electricity production. Any wind system that would be constructed in conjunction with Federal new construction or major renovation projects is likely to be much smaller. Distributed energy wind projects, or projects designed to deliver electricity to a facility directly, can use a range of turbine sizes but often only consist of one or two turbines.
This overview is intended to provide specific details for Federal agencies considering geothermal heat pump technologies as part of a new construction project or major renovation. Further general information is available from the U.S. Department of Energy (DOE) Office of Energy Efficiency and Renewable Energy (EERE) Wind Energy Basics.
Description
A typical wind turbine is made up of several key components including:
- The nacelle:
- Bed plate
- Gearbox
- Generator
- Controller and power boxes.
- Rotor:
- Hub
- Blades.
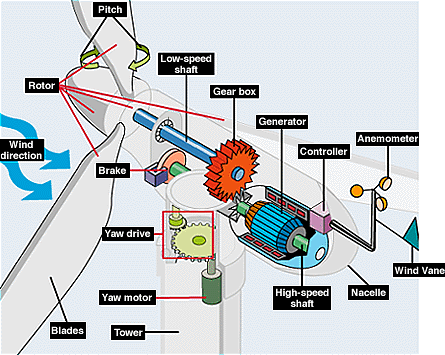
Typical wind turbine components
The nacelle houses all of the internal parts of the turbine including the bed plate, the gear box, the generator, the controller, the yaw drive, and the hydraulics. The bed plate directs the aerodynamic forces to the tower, but is not associated with the generation of power. The gearbox steps up the revolutions from the low-speed shaft and the hub, which is attached to the turbine blades to the high speed shaft of the generator (1,800 revolutions per minute). Not all wind turbines include gearboxes, some may use a different "direct drive" generator design. A generator is used to convert this shaft power to electricity. The wind turbine's controller monitors many system parameters and, depending on the wind turbine design, continually adjusts the wind turbine's blade pitch angle for optimum rotational speed and appropriate lift-to-drag ratios at varying wind speeds.
The rotor includes the elements of the turbine that rotate in the wind, including the hub, the blades, and for pitch-regulated wind turbines, the blade pitch mechanisms and bearings. It is through the rotor that the wind energy is transformed into mechanical energy that turns the main shaft of the wind turbine. The blade root is bolted to the hub. Most horizontal axis wind turbines have three blades that convert the push of the wind into a circular motion.
Current Types and Costs of Technology
There are a variety of wind turbine designs for converting wind into electricity. Wind turbines should be designed to be equally robust and efficient, lightweight and sturdy. Although the concept of wind energy is simple, the design of turbines to meet these criteria is very complex. Most modern wind turbines use aerodynamic lift principles to convert wind power into a rotational force.
The two main types of wind turbines in the industry today are named after their orientation: horizontal axis wind turbines and vertical axis wind turbines.
Horizontal Axis Wind Turbines
Horizontal axis wind turbines are by far the most common type of turbine and account for almost all of the installed wind capacity in the United States. These turbines can range in size from 100 watts (W) to 5 MW. The wind industry has several distinct sectors, each with its own technology characteristics and development and deployment strategies:
- Offshore utility-scale wind. Typically above 3 MW, these wind turbines are currently installed in shallow waters of 30 m, or approximately 98 ft., or less and in large groups at sites with good wind resources. Currently no offshore wind installations have been installed in the United States. However, several gigawatts (GW) of capacity have been installed in Europe and plans are in place for the first test deployment of U.S. offshore wind farms, located primarily in the North East and Great Lakes.
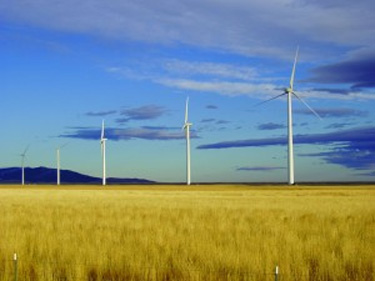
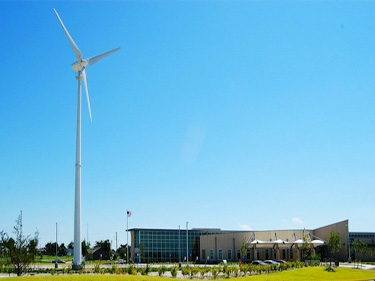
The Judith Gap Wind Energy Center in Montana is comprised of 90 GE 1.5–MW turbines, for a total capacity of 135 MW.
The Kiowa County Memorial Hospital in Greensburg, Kansas is expected to achieve annual energy cost savings of more than 40%, due to features like the on-site, grid-tied, 50–kW wind turbine that will generate 220,000 kWh annually.
-
Utility-scale wind. Normally installed in large groups, or wind farms, and at sites with a very good wind resource, these 1 to 3 MW wind turbines inject clean green energy onto the utility grid via transmission lines. Most of the wind industry growth is due to this sector. A total of 37 states in the United States have installed utility-scale wind power. Turbines used in wind farms for commercial production of electric power are three-bladed and actively directed into the wind.
-
Electric utilities add wind power to their power supply portfolios as a clean and competitively-priced source of electric generation. Investor-owned utilities, municipal public power utilities, and rural electric co-operatives all have a unique role in bringing wind power to their retail customers.
-
Community-scale wind. These wind turbines are 100 kilowatts (kW) and larger and are installed individually or in smaller groups. They are connected to the electricity grid on the customer side of the utility meter and are used to reduce the amount of electricity the customer—or community, school, or company—purchases from the utility.
This scale of wind project is less common, since it depends on a close interaction between the utility customer and the renewable energy developer, and it requires interconnection with the utility. The cost per kilowatt of installed wind energy is higher in community-scale projects; the value of electricity from these projects is also higher. Because the projects offset purchases from the utility, they compete with the retail price of electricity, as opposed to the wholesale price of power paid to utility-scale projects. - Residential-scale wind. These wind turbines are 1 to 100 kW in size and are normally installed at a home or small business on the customer side of the utility meter to reduce the amount of electricity purchased from the utility. The United States also leads the world in the production of small wind turbines, which are defined as having rated capacities of 100 kW or less.
There are tens of thousands of smaller turbines used in applications to provide ranchers, farmers, homeowners, and small business owners with the ability to generate their own electricity, and to partially or wholly replace electricity from the grid. Significant research and development has been undertaken in the past decade to improve the performance and lower the cost of these smaller turbines.
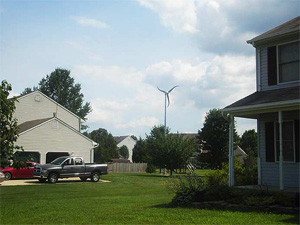
Southwest Windpower's 1.8–kW Skystream wind turbine is designed for residential application.
Each of these sectors of the wind industry essentially have different technologies. They may all be called by the same name, but they have profound, fundamental differences, assessment methods, economics, environmental considerations, and other factors that drive each sector.
Vertical Axis Wind Turbines
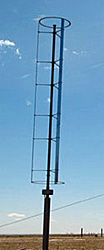
The Mariah Windspire 1–kW wind turbine installation at the National Wind Technology Center. An example of a Darrieus blade, this turbine is a part of the Small Turbine Independent Testing program.
Vertical axis wind turbines have been in use for many years with many different design variations. However, all the different designs can be categorized into two basic design types: Savonius rotor and Darrieus blade.
The Savonius rotor is a drag type of rotor that is pushed by the wind and is much less efficient than horizontal axis wind turbines. This turbine provides a high torque and is more effective at high wind speed locations. Some Savonius turbines are designed for installation in remote and harsh climates, where a small amount of power is needed and other alternatives do not work well. Therefore, its high cost of energy is not a limiting factor. Unfortunately, this technology currently lacks a clear track record for mainstream applications. This type of turbine can be more suitable for specific applications such as sign or buoy lighting.
The other most common type of vertical axis wind turbine is the Darrieus blade. The turbine consists of two or more airfoil blades which are attached to the top and bottom of a rotating vertical shaft. The Darrieus rotor utilizes the lift principle and thus is capable of achieving aerodynamic efficiencies similar to that observed in horizontal axis wind turbines.
The simple limiting factor of all vertical axis wind turbines is that the central rotating shaft must remain rigid and cannot develop a wobble, which may be difficult if the turbine is expected to operate with limited maintenance over its design life. To address this issue, the turbines must either incorporate a very solid lower barring or the top of the unit must be fixed with an upper barring and guy wires. This typically limits the size of the turbine and/or its installation location.
Horizontal Axis vs. Vertical Axis Wind Turbines
In 2003, Global Energy Concepts completed a study comparing horizontal axis wind turbines and vertical axis wind turbines. The main conclusions were:
-
Vertical axis wind turbines will be 15% to 25% less efficient than horizontal axis wind turbines and will capture less energy for the same swept area.
-
Vertical axis wind turbine savings could come from lower maintenance costs; however, these savings are unlikely to balance the lower energy capture and higher initial rotor costs.
-
Both types of machines are susceptible to fatigue loads. Horizontal axis wind turbines are more sensitive to turbulence, while vertical axis wind turbines will suffer from resonant conditions at variable wind speeds.
In addition to being less efficient, vertical axis wind turbines are usually located closer to the ground in more directionally variable locations than horizontal axis wind turbines. As noted before, taller towers provide far better access to more energetic and productive winds. Taller towers also allow for the turbine to gain clearance above obstacles on the ground, such as existing trees and buildings that cause turbulence in the wind, which leads to decreased production and increased wear on the wind turbines.
Application
The following criteria are pre-conditions to satisfy before thoroughly assessing the wind potential at a particular site:
-
Obstacles and wind shear. Selecting a wind turbine project site is crucial to its long-term performance. The wind energy project should be placed in a location with a wide and open access to the prevailing winds. Obstacles diminish the energy available in the wind and increase turbulence, both of which decrease the turbine's energy production. Ground roughness also affects wind speeds; the more pronounced the roughness of the terrain, the more the wind will be slowed down.
-
Proximity to distribution line interconnection. For all scales of wind projects, the electrical connection from the wind project to the point of interconnection is a factor. Specifically for small projects, this cost can be significant. Therefore, the distance and the cost of the conductor should be minimized where possible. For larger projects, this factor becomes far less important. The choice of whether to use buried or above-ground conductors is generally based on the standard practices where the project is to be sited. Small turbines can largely be interconnected directly into a building or local electrical distribution system while larger turbines will require interconnection to more established electrical transmission systems. When considering potential wind turbines sites at a Federal facility, the electrical interconnection point will always be a critical early determination.
-
Reasonable road access. For smaller turbines, road access is typically not an issue. For Federal agencies considering a wind turbine project using utility-scale wind turbines, adequate access for large wind turbine component shipments (tower sections and blades) and heavy construction equipment (cranes) needs to be fully investigated. A typical site road width requirement for utility-scale wind turbines is 18 feet. Turning radius and slope for existing roads should be fully investigated long before components are shipped to the site.
Due to the cost of temporary or permanent road enhancements, and when considering larger projects, wind developers look for roads that are usable "as is" for getting close to the general wind farm site. Generator-specific road construction for each turbine site is a standard facet of the construction process. - Siting of the turbine. The siting of the turbine on the facility property is important not only for its access to the wind and interconnection points, but also for the impacts of the turbine on the facility and surrounding areas. Wind turbines make noise, which is mitigated somewhat by the wind itself but also by distance from the turbine. Best practice also places the turbine such that the "drop zone," or circumference around the turbine that equals the turbine height, is clear of inhabited facilities. The turbine should be assessed for noise and other impacts, and sited to ensure that there is no disruption to facility occupants or surrounding neighbors.
Economics
Wind power produces energy only when the wind blows. For an on-site wind energy project to take advantage of the electricity produced from the turbine, the project needs access to favorable utility policies, particularly interconnection requirements and net metering. A Federal agency should choose a wind turbine that has an expected energy production figure that aligns with the energy load of the facility during the time period designated by the net metering rules. Enabling the wind project to offset full retail rate for all of the energy produced will enhance the project's economic payback.
The economic cost to produce electricity with wind varies considerably with the turbine size and the wind resource. Assuming a Class 3 wind resource, which is a lower-level but usable wind resource, the rough range of electricity produced is provided in the following table.
Cost of electricity produced for different turbine size ranges in a class 3 wind regime.
Turbine Size | Cost of Electricity Produced per kilowatt-hour (kWh) |
---|---|
1 to 20 kW | $0.20 to 0.40/kWh |
20 to 200 kW | $0.12 to 0.20/kWh |
200 to 1500 kW | $0.08 to 0.15/kWh |
1.0 to 3.0 MW (1 to 5 turbines) | $0.07 to 0.12/kWh |
1.0 to 3.0 MW (50 to 500 turbines) | $0.05 to 0.09/kWh |
The economic analysis of a wind project for a Federal agency should include an examination of the relationship between the retail cost of electricity purchases and the expected energy production for the wind resource at the turbine site. Wind turbines are most cost effective where the average wind speed is high, where the competing energy costs are high, or where a combination of both are true. Sites with a high retail rate for electricity but less wind available can achieve better economics than sites with more wind available, but a very low cost of avoided electricity.
Large wind farms of 100 to 500 MW have been driving the industry due to their economies of scale. Conversely, a Federal site with only one or two turbines would not achieve the same economies of scale. Thus, the resulting cost for wind energy should be expected to be higher than the wind farm industry norm.
There are many types of incentives which can appeal to the parties involved in a wind turbine project depending upon their role, available resources, and the impact of the incentive on their resources. Incentives, such as the production tax credit and accelerated depreciation, have been strong drivers for equity partners in wind farms. Net metering has been an incentive that appeals to the local owner of smaller projects that offset retail-rate power. The Database of State Incentives for Renewables & Efficiency (DSIRE) has more information on available incentives for wind energy.
Federal agencies often cannot take advantage of many of the subsidies using standard financing protocols. If these incentives make the difference in life-cycle cost effectiveness for the project, the agency should consider other forms of renewable energy project funding.
The Federal Energy Management Program (FEMP)'s Guide to Integrating Renewable Energy in Federal Construction has more information on:
- Interconnection requirements and net metering
- Renewable energy project funding
Renewable Energy Certificates
Renewable energy projects generate additional value in the form of renewable energy certificates. For wind projects, renewable energy certificates can add value to the project, but Federal agencies must retain the certificates to get credit for the renewable energy use. Although wind renewable energy certificates are not valued as highly as other types, there may still be an opportunity to trade a wind renewable energy certificate for one with a lower value, such as one from a landfill methane-type project, and use the net difference to help finance the project.
FEMP's Guide to Integrating Renewable Energy in Federal Construction has more information on renewable energy certificates.
Assessing Resource Availability
Wind is air with high kinetic energy that can be transformed into useful work via wind turbine blades and a generator. Overall, wind is a broadly available resource that can generate competitively-priced electricity in many regions.
Wind Characteristics
Wind speed varies with the season, time of day, and weather events. According to the Danish Wind Industry Association, roughly 1% to 2% of the sun's energy that strikes the earth is transformed into wind. Collected wind data focuses on two primary considerations: average annual wind speed and a frequency distribution of the wind at various speeds. The wind speed at any given time determines the amount of power available in the wind.
Wind Classifications and Wind Maps
Wind power is related to the cube of the wind speed (V³). This relationship is important because as the wind speed doubles, the power increases by a factor of eight (2³ = 8). Thus, small changes in speed can result in significant differences in energy production.
Wind quality, or clean flowing wind, is just as important as wind quantity, or speed. Turbulence in the air decreases the electricity generated from the wind and also creates trouble for the equipment—by increasing maintenance and decreasing life expectance. In addition, wind speed decreases behind an obstacle, such as a building or trees, and so it is extremely important to account for local wind obstacles in the prevailing wind direction near the turbine to make accurate energy output predictions.
Appropriate tower height for a wind turbine is essential for optimal performance. Taller towers provide access to higher wind speeds and lower turbulence, so typically, the taller the tower, the higher the electricity production from a turbine. It is important, however, to achieve a balance between the increase in electricity and the increased costs associated with a taller tower.
There is a wind classification system that has been developed to quantify wind resources using a term called wind power density, which defines the wind power available per square meter of rotor area.
Classes of wind power density
Wind Power Class | Wind Power Density at 10 m (33ft) | Wind Speed | Wind Power Density at 30 m (98 ft) | Wind Speed | Wind Power Density at 50 m (164 ft) | Wind Speed |
---|---|---|---|---|---|---|
W/ m² | m/s (ft) | W/m² | m/s (ft) | W/ m² | m/s (ft) | |
1 | 100 | 4.4 (9.8) | 160 | 5.1 (11.4) | 200 | 5.6 (12.5) |
2 | 150 | 5.1 (11.5) | 240 | 5.9 (13.2) | 300 | 6.4 (14.3) |
3 | 200 | 5.6 (12.5) | 320 | 6.5 (14.6) | 400 | 7.0 (15.7) |
4 | 250 | 6.0 (13.4) | 400 | 7.0 (15.7) | 500 | 7.5 (16.8) |
5 | 300 | 6.4 (14.3) | 480 | 7.4 (16.6) | 600 | 8.0 (17.9) |
6 | 400 | 7.0 (15.7) | 640 | 8.2 (18.3) | 800 | 8.8 (19.7) |
7 | 1000 | 9.4 (21.1) | 1600 | 11.0 (24.7) | 2000 | 11.9 (26.6) |
Evidence of Significant Wind
The extent of the wind resource is a critical parameter for a successful project. At the initial stage, the cursory investigation can include examination of wind maps, observation of trees flagging at or near the site, conversations with locals familiar with the wind resource, and the presence of wind farms. The WindExchange program maintains a website with high quality wind maps.
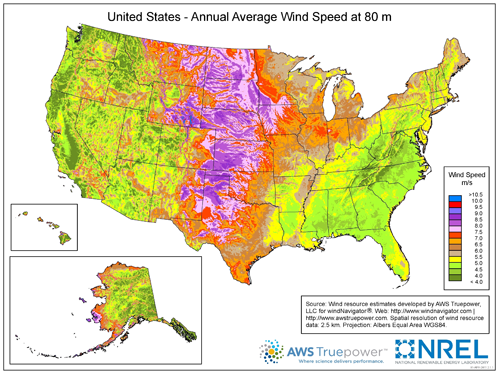
U.S. wind speed map at 80 m
For small wind projects, wind maps or available wind data may be sufficient for determining the relative economic feasibility of a site. One source for publically available climate data is the Utah Climate Center.
Understanding the prevailing wind direction is especially important when siting small projects in the vicinity of obstacles such as trees or buildings. Wind roses, which graphically represent distribution of wind speed and direction, provide this important insight. Production results from nearby wind turbines can reveal information about local wind conditions.
For community-scale projects, one viable approach is to purchase a representative long-term data set or a typical wind year from a commercial entity. New and developing technologies such as SODAR (sonic detection and ranging) and LIDAR (light detection and ranging) are demonstrating that even short-term, on-site resource measurement can increase confidence in the local wind resource.
Wind maps indicate the general wind resource in an area, but generally do not provide the data necessary for development of investment-grade annual energy estimates that are especially important if financing is required for a project. As a project develops, the industry convention for planning larger, utility-scale wind projects typically involves collecting and analyzing on-site wind data at or near the proposed hub height and location of the potential wind turbine for at least 12 months. The collected data is then correlated to a nearby, long-term wind speed reference data set for wind resource verification. This process increases the level of confidence in estimated annual production estimates to the degree required for financing larger projects.
Although this level of on-site data collection is often not necessary for smaller projects, Federal agencies considering the purchase and installation of community-scale wind projects should ensure that the feasibility study includes a full wind resource assessment so the economic analysis can be robust and, if needed, financeable.
Design Considerations
When planning and designing wind technology systems, it is important to do all of the following:
- Provide area or wall space near electrical equipment for wind turbine disconnect and related equipment
- Avoid mounting turbines on, or too close, to buildings
- Locate turbine on highest, unobstructed area of property and far enough from populated areas to reduce noise concerns
- Locate turbine so that nothing of value is within fall zone of turbine, blades, and guy cables (if applicable)
- Locate turbine near enough to electrical equipment, however, so that trenching and cable costs do not become excessive
- Consider the wind wakes and turbulence created by all buildings, trees, and structures on property, and use tall enough tower to avoid "shading" of turbine
- Oversize the electrical panel that will receive solar power to accommodate the sum of utility power plus solar power, as per code requirement as the sum of wind and grid input breakers can never exceed 125% of the panel rating. Only the panel needs to be oversized, not the entire service to the building.
Procurement Considerations
Many agencies and contracting officers within these agencies are unfamiliar with the procurement of a wind turbine. And although they may be considered experts in contracting, they may not necessarily be proficient at specifying, selecting, soliciting, and purchasing a robust, reliable, and economic wind turbine that will serve the agency well for its useful life (~20 years and beyond). Due to the current wide variety of wind turbines and the lack of strong and enforced international standards for the design and manufacturing of wind turbines, especially small turbines, extreme care should be taken in identifying reputable wind turbine equipment suppliers.
Any procurement of wind turbines should be done with the advice of a wind expert. In all cases, turbines should have some sort of independent certification from a recognized certification agent. Additionally, the turbine, and its manufacturer, should have a clear and demonstrated deployment track record. It is also important to note that the installation of the turbine should be conducted by an organization with a solid, demonstrated history—even the best technology implemented incorrectly will not likely meet customer expectations.
System design and installation are also critical. Although the project team can use a range of standard contractors and electricians, the system should be designed and installed by wind turbine experts with significant demonstrated experience in wind turbine design, construction, and verification.
Operation and Maintenance
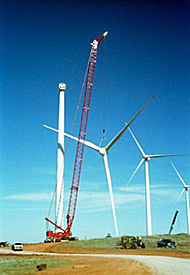
Red Hills Wind Farm is located 10 miles north of Elk City, Oklahoma in Roger Mills and Custer counties
Wind turbines require regular maintenance. Manufacturer warranties typically only cover the first 2 to 5 years. Most manufacturers of utility-scale wind turbines offer long-term (10-year) integrated service plans that provide qualified service during the financing period. Where these services are not available, professional wind turbine maintenance contractors, or windsmiths, are recommended after the warranty period. The corresponding figure shows large wind turbines that could be considered for a Federal site with sufficient land.
Operation and maintenance programs for wind turbines should include consider the following:
- Frequency and time involved in routine maintenance
- Special skills or training required to service equipment
- Costs associated with maintenance
- Discussion of controls and remote monitoring
- List of components which need to be refurbished or replaced during system life.
Special Considerations
When integrating wind technology into construction projects, it is important to consider the issues related to interconnection, the National Environmental Policy Act (NEPA), aviation and radar impacts, and other applicable issues.
Interconnection
As with other electricity generating technologies, wind turbines need to be connected to the electricity grid unless they are powering a remote facility. In the case of Federal new construction or major renovation projects, the turbines will most likely need to be interconnected to the local utility at or near the point that the entire facility is connected to the utility. Rules vary by state and locality as to the size and type of technology that can be interconnected, so this needs to be reviewed for the facility site as soon as the location is known.
FEMP's Guide to Integrating Renewable Energy in Federal Construction has more information on key policies for renewable electricity.
National Environmental Policy Act
Construction projects for Federal agencies must follow the strict guidelines required by NEPA. Early review of noise and "flicker" (the effect of sunlight passing through the fan blades) of the turbine as well as permitting and zoning issues can determine if there is a site with good wind resource capable of minimizing the turbine's impact on the facility and surrounding neighbors. The NEPA process helps to inform nearby neighbors and communities of any significant wind development activities at the Federal site. Because of the visible nature of wind projects and a lack of understanding in most communities, Federal agencies should keep the public well informed of pending wind projects as part of the public information on the project. Having upfront, detailed information on visibility, noise, flicker, and other perceived impacts of the turbine can help reduce the community's concerns.
The NEPA process can also address impacts at nearby historical properties and review the impact of any potential turbine on protected wildlife or migratory birds. Most of these issues, especially wildlife impacts, are limited with small turbines under 100 kW; however larger turbines are more likely to encounter this type of review. Ensure that the NEPA compliance official on the project is trained on wind issues, as most reviewing officials at various agencies will have very little experience with wind turbines, and therefore, little understanding of their impacts.
Before a Federal agency commits significant resources, there are a few red flags that might preclude wind development at a later stage. For example, endangered species that frequent the area, or a wilderness area designation could become environmental concerns that might prevent a site from passing the first cut of site selection. Proximity to an airport could limit the potential height of a turbine. Early in the design process of a Federal construction project, the agency should have a feasibility study that reviews environmental and permitting issues related to wind turbine development.
FEMP's Guide to Integrating Renewable Energy in Federal Construction has more information on conducting a feasibility study.
Aviation and Radar Impacts
All wind turbines with a maximum rotor tip height of more than 61 m (~200 ft), are subject to notification and approval requirements by a series of Federal agencies with responsibilities for airspace and flight operations. These agencies are listed in the following table.
Type of radar or operation and the regulating authority.
Type of Radar or Operation | Federal Agency |
---|---|
Defense radar and military training routes | Department of Defense |
Next Generation Weather Radar Program | Departments of Commerce, Defense, and Transportation |
Long-range radar and airport runways | Federal Aviation Administration |
Other Considerations
When they are well-planned and sited correctly, large wind turbines can produce a large amount of electricity with no emissions and provide an agency with a significant reduction in greenhouse gas emissions.
Additional Resources
Publications
Websites
- American Wind Energy Association offers information on federal and state wind energy policy and up-to-date news on the issues most relative to the wind industry.
- National Wind Technology Center identifies research capabilities and current projects being pursued at the National Renewable Energy Laboratory's National Wind Technology Center.
- DOE's Wind Program provides information from the national laboratories that are conducting research on wind and water technology as well as the U.S. Department of Energy "20% Wind Energy by 2030" Report.
- WindExcahnge is the DOE Wind Program's platform for disseminating credible information about wind energy.
Resource Assessment Tools
- NREL's Wind Research program has information on Resource Assessments